When a magnetar in the Milky Way spewed a burst of colossally powerful radio waves in 2020scientists finally had concrete evidence to determine the origin of rapid radio bursts.
A stunning new study has clarified the mechanism. By studying the sparkling light of a rapid radio burst detected in 2022, a team of astronomers traced its source to the powerful magnetic field around a magnetar, in a galaxy 200 million light years away.
This is the first conclusive evidence that fast radio bursts can emerge from the magnetospheres of magnetars.
“In these neutron star environments, the magnetic fields are really at the limits of what the Universe can produce,” says astrophysicist Kenzie Nimmo from the Massachusetts Institute of Technology (MIT).
“There has been much debate about whether this bright radio emission could even escape from this extreme plasma.”
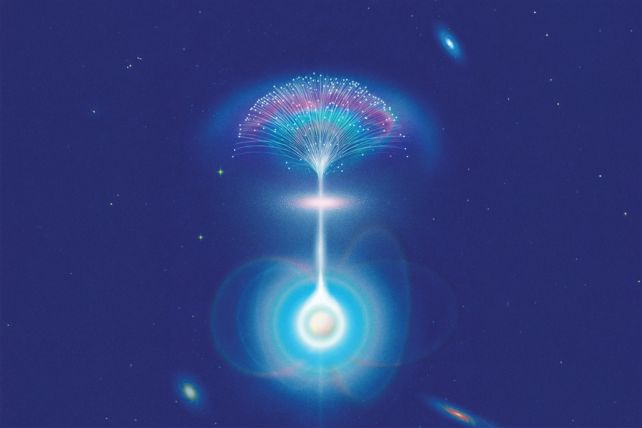
Fast radio bursts (FRBs) have intrigued scientists since their appearance. first discovered in 2007. These are, as their name suggests, extremely brief radio broadcasts, lasting only a few milliseconds. They are also extremely powerful, sometimes releasing more energy than 500 million suns in this brief moment.
FRBs are difficult to study because most of the time they only erupt once. This makes them impossible to predict and difficult – but not impossible – to trace back to a source. A number of point FRBs have been traced in galaxies located millions or even billions of light years of space-time.
Astronomers can also look at the properties of radio light, such as its polarization, to determine what type of environment it passed through on its way to Earth. The types of stars that can emit FRBs remain largely a mystery, but growing evidence increasingly involves magnetars.
Magnetars are particularly unusual neutron stars, which themselves are the extremely dense core remnants left after a massive star goes supernova. But magnetars have much stronger external magnetic fields than ordinary neutron stars – about 1,000 times stronger. These are the strongest magnetic fields in the Universe.
frameborder=”0″allow=”accelerometer; autoplay; clipboard-writing; encrypted media; gyroscope; picture in picture; web sharing” referrerpolicy=”strict-origin-when-cross-origin”allowfullscreen>
“Around these highly magnetic neutron stars, also known as magnetars, atoms cannot exist: they would simply be torn apart by magnetic fields.” says physicist Kiyoshi Masui from MIT.
“What’s exciting here is that we see that the energy stored in these magnetic fields, close to the source, twists and reconfigures itself in such a way that it can be released as radio waves that we We can see to the other side of the Universe.”
To trace the origin of an FRB, Nimmo and his colleagues studied a property known as scintillation in an event known as FRB 20221022A, first detected in 2022 and later traced to a galaxy located 200 million light years away. Scintillation is what makes stars twinkle – the distortion of the path of light as it travels through gases in space. The longer the distance traveled, the stronger the flicker.
FRB 20221022A is a pretty standard standard, as far as FRBs go. It was moderately long, about 2 milliseconds, and moderately powerful. This makes it an excellent case study for trying to understand the properties of other FRBs as well.
A complementary document study the polarization of light of FRB 20221022A – the degree to which the orientation of its waves is twisted – found an S-shaped angle oscillation consistent with a rotating object, a first for an FRB. This suggests that the signal came from very close to the rotating object.
Nimmo and his colleagues found that if they could determine how scintillating FRB 20221022A was, they could calculate the size of the region where it came from. Light from the FRB showed strong scintillation, leading researchers to the gas region that distorted the signal. Using this gaseous region as a lens, they narrowed the FRB’s source to within 10,000 kilometers (6,213 miles) of its magnetar source.
“Zooming in on a region of 10,000 kilometers, at a distance of 200 million light years, is like being able to measure the width of a DNA helix, which is about 2 nanometers wide, on the surface of ‘an object. the Moon,” Masui says. “There’s an incredible range of scales involved.”
This is the first conclusive evidence that extragalactic FRBs can originate from the magnetosphere of highly magnetized neutron stars. But it’s much more than that. The techniques used by the team show that scintillation can be a powerful probe for other FRBs, so astronomers can try to understand how diverse they might be – and whether other types of stars could also belch the powerful eruptions.
“These explosions are still happening” Masui says. “There can be a lot of diversity in how and where they occur, and this scintillation technique will be very useful in helping to unravel the different physics that are causing these bursts.”
The research was published in Nature.